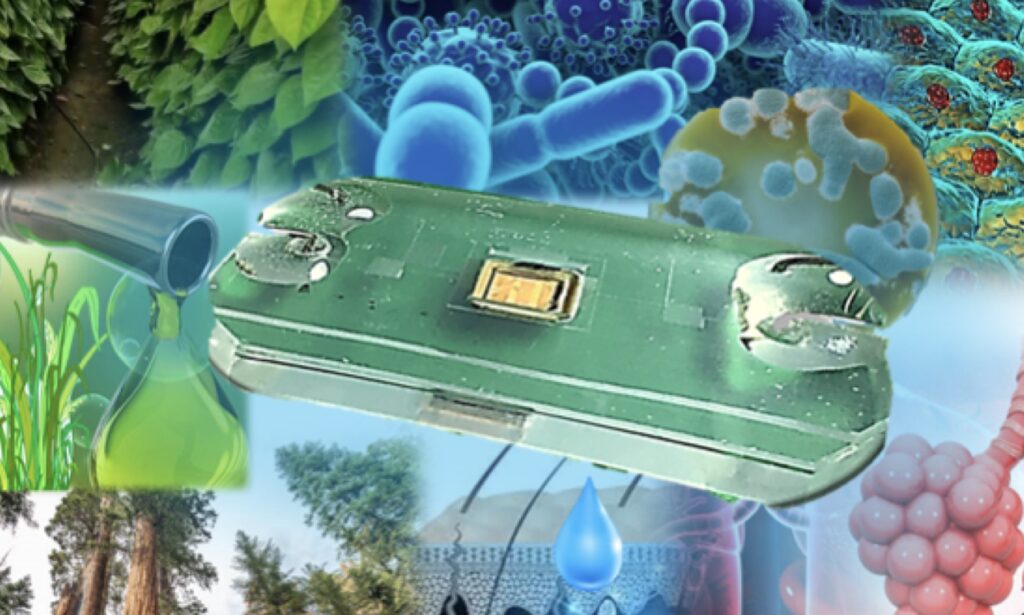
The Science
The structure of cellulose is predominated by highly-ordered rope-like structures known as ‘fibrils,’ making this biopolymer an intriguing feedstock for manufactured nanomaterials with the potential for impressive mechanical properties. Additionally, cellulose depolymerization yields glucose, which can be used to produce biochemicals and biofuels. But the intra- and intermolecular hydrogen bonds that give cellulose fibrils their impressive mechanical properties also reduce the accessibility of enzyme interaction sites, limiting the efficiency of the enzyme-driven hydrolysis steps needed to process cellulose biomass into usable products. This work builds upon prior research by other teams to better understand the dynamics of enzyme-driven cellulose hydrolysis by introducing a breakthrough open microfluidic device. The device enables real-time, in situ characterization of these important biochemical processes, that were previously thought impossible to perform, when coupled with non-destructive, synchrotron-generated infrared light. The developed technology can easily be extended to other biomolecular systems of interest, to provide new insight through real-time investigations at high spatial resolution.
The Impact
With the recent introduction of closed microfluidic systems into synchrotron-based radiation Fourier-transform infrared (SR-FTIR) spectroscopy techniques, researchers have new options for non-destructively probing sub-cellular systems at high spatial and temporal resolutions. FTIR provides insights into chemical bonds that typify systems, and changing patterns of chemical bonds, with the potential to elucidate the biochemical dynamics of living systems in response to changing stimuli and other environmental cues. This work took microfluidic methods one step further by employing a novel open microfluidic device, which enables on-demand access to the sample environment during infrared analysis while autonomously maintaining the ultra-thin liquid films required for efficient infrared analysis. The breakthrough results serve as a key proof-of-concept for the technique, with the potential to enable a new generation of scientific discoveries — especially for researchers hoping to characterize the physical and chemical properties of biological systems in situ and in real time. The study also sheds new light on the spatiotemporal dynamics of enzymatic hydrolysis in cellulose, an important step toward harnessing this important biofeedstock toward a circular bioeconomy.
Summary
Various studies and scientific techniques have provided important insight into enzymatic interactions with cellulose biomass. Even so, the role of the cellulose supramolecular structure (its fibrils and hydrogen bonds) in the efficiency of such enzymatic interactions remains unclear. The best way to gain deep insight into these processes is through the use of in situ, time- and spatially-resolved characterization techniques, which is why researchers in this study coupled a novel open-channel, high-density capillary microfluidic device with existing SR-FTIR techniques.
Until now, the enzymatic hydrolysis of cellulose was a challenging biochemical process to study, in part due to limitations in researchers’ ability to carefully control, and observe, the sample environment (such as the reactive fluids or relative humidity). The technique used in this work overcomes these challenges, through several approaches. First, the exceptional brightness of the synchrotron infrared light source supports real-time data capture. Second, the ‘open’ nature of the microfluidic device allows for on-demand access to the sample environment, with autonomous, precise control of relative humidity, even as more reactive fluid is added. As a result, the approach supports a sustainable technique for detecting key spectral signals as the biochemical reaction of interest proceeds within the microfluidic sample environment — an approach that could be extended across a range of systems and sample types to reveal insights previously thought inaccessible to science.
In this work, the time-resolved depletion of algal cellulose by a purified cellulose-degrading enzyme was tracked by monitoring a set of absorption peak intensities associated with the glycosidic bond — the covalent bond that joins glucose monomers within the cellulose structure. The results demonstrated high spatial heterogeneity of the enzymatic process within the sample, found to be consistent with the notion that fibrillar regions containing large amounts of intra- and intermolecular hydrogen bonding are able to resist enzymatic processes. This insight will help researchers devise new approaches for cellulose biomass processing, with the ultimate goal of developing a range of manufacturing applications toward a more sustainable and circular bioeconomy. The collaboration included researchers from the Department of Biological and Agricultural Engineering at the University of California, Davis, and the Lawrence Berkeley National Laboratory (LBNL). LBNL is home to the Berkeley Synchrotron Infrared Structural Biology (BSISB) program, funded by DOE-BER, and the Advanced Light Source, a DOE Office of Science user facility. Advances in closed and open high-density microfluidic technologies developed through BSISB-led R&D initiatives can directly benefit BSISB users who require controllable sample environments or reaction conditions, as well as in situ monitoring at high spatial resolution.
Funding Acknowledgements
This work was supported by the U.S. Department of Energy, Office of Science, Office of Biological and Environmental Research under Contract Nos. DEAC02-05CH11231 and DE-SC0019228. Co-author JD Nill was supported in part by the U.S. Department of Energy, Office of Science Graduate Student Research program under Contract No. DE-SC0014664, and co-author SR Narayanasamy was supported in part by the LLNL Postdoctoral Career Development Grant.
Related links
- Berkeley Lab News: Watching the Enzymes that Convert Plant Fibers Into Simple Sugars
- ALS Science Highlight: Tracking the Breakdown of Cellulose at the Micron Scale
- UC Davis News: Research Sheds Light on Using Agricultural Residues for Fuels, Chemicals
- Eurekalert: Watching the enzymes that convert plant fibers into simple sugars
References
Jeoh T, Nill JD, Zhao W, Narayanasamy SR, Chen L, and Holman HYN. Spatiotemporal dynamics of cellulose during enzymatic hydrolysis studied by infrared spectromicroscopy. Green Chemistry (2023). (https://doi.org/10.1039/D3GC03279E)